Abstract
Stereoisomerism significantly influences the biological activity, environmental behavior, and toxicity of pesticide molecules. Approximately a quarter of registered pesticides are chiral, containing one or more chiral centers and existing as enantiomers with differing biological properties—one often highly effective, the other less active or even harmful. Despite this, most commercial pesticides remain racemic mixtures, leading to inefficiencies and ecological concerns. This article explains key stereochemistry concepts in pesticide chemistry, detailing geometric and optical isomers and illustrating their impact through examples. Emphasizing the need for enantioselective formulations, it highlights how optimizing pesticide composition can enhance efficacy while reducing environmental risks and non-target toxicity.
INTRODUCTION TO STEREOISOMERS
Imagine you are looking at two objects that seem identical at first glance, but when you try to place one on top of the other, they don’t match perfectly. This is the idea behind stereoisomers—molecules that have the same atoms and the same connections between them but differ in how those atoms are arranged in space (spatial arrangement).
Types of Stereoisomers
Stereoisomers can be broadly categorized into two major classes: geometric isomers and optical isomers, with optical isomers further divided into enantiomers and diastereomers.
Geometric Isomers (Cis-Trans or E/Z Isomers)
Geometric isomerism happens when a molecule has restricted rotation, such as around a double bond or within a ring structure. This means the attached groups can be locked in different positions:
Cis isomer: The important groups are on the same side.
Trans isomer: The important groups are on opposite sides.
In systematic nomenclature, when there are more complex groups attached, we use the E/Z system, which follows priority Cahn-Ingold-Prelog rules to name the isomers.
Optical Isomers and Chirality
Optical isomerism is a result of chirality. A molecule is chiral if it has a structure that makes it impossible to superimpose onto its mirror image—just like how your left and right hands are mirror images but not the same. The most common reason for chirality in molecules is the presence of a chiral carbon (a carbon atom bonded to four different groups).
Enantiomers: Non-Superimposable Mirror Images
Enantiomers are a pair of optical isomers that are non-superimposable mirror images of each other.
They have the same physical properties except when interacting with polarized light or with other chiral substances.
One enantiomer rotates light in a clockwise direction (dextrorotatory, or (+)), while the other rotates it counterclockwise (levorotatory, or (-)).
To further classify enantiomers, chemists use the R- and S- system, which follows the Cahn-Ingold-Prelog priority rules:
R- (Rectus, Latin for "right"): If the sequence of priority groups rotates clockwise when viewed from the lowest priority group, the molecule is labeled R.
S- (Sinister, Latin for "left"): If the sequence of priority groups rotates counterclockwise, the molecule is labeled S.
For example, a pesticide with an S-enantiomer might be much more effective than its R-enantiomer, or vice versa.
Diastereomers: Stereoisomers That Are Not Mirror Images
Diastereomers are stereoisomers that are not mirror images of each other.
They usually occur when a molecule has more than one chiral carbon, leading to multiple possible arrangements in space.
Unlike enantiomers, diastereomers can have different physical and chemical properties, such as solubility and reactivity.
A simple way to understand chirality is to compare chiral and achiral molecules:
If a molecule’s mirror image can be placed on top of the original so that all parts align perfectly, it is superimposable and therefore achiral.
If the mirror image does not match up in every detail, the molecule is non-superimposable and chiral.
WHY IS STEREOCHEMISTRY IMPORTANT IN PESTICIDES?
In pesticide chemistry, stereoisomerism plays a major role in how effective a pesticide is and how it interacts with the environment. Many pesticides exist as pairs of enantiomers, where:
One enantiomer (e.g., S- or R-) may be highly active against pests.
The other enantiomer may be inactive or even cause unwanted environmental effects.
At present, approximately a quarter of registered pesticides are chiral, meaning they contain one or more chiral centers and exist as enantiomers. This proportion is expected to increase as more chiral pesticide structures are developed. In China alone, more than 40% of pesticides used are chiral.
Even though enantiomers have very similar physicochemical properties, their biological activity can differ significantly, making chiral pesticides enantioselective. A striking example is the triazole fungicide triadimenol, which has two chiral centers and four enantiomers. Among them, S,R-triadimenol is 1000 times more active than the other three enantiomers.
Typically, only one enantiomer has the desired biological activity, while the other enantiomers may be ineffective or even harmful. Despite this, 93% of chiral pesticides are still used as racemates—mixtures of both enantiomers—allowing for unintended environmental and biological consequences.
Beyond toxicity differences, studies have shown that chiral pesticides differ in their effects on:
Neurotoxicity, endocrine disruption, reproductive and developmental toxicity, genotoxicity, immunotoxicity.
In addition, chiral pesticides degrade at different rates in the environment, further complicating their impact. Research has demonstrated that using only the active enantiomer instead of the racemate could reduce environmental damage and enhance pesticide efficiency.
Thus, it is crucial to systematically study the health risks of different pesticide enantiomers on non-target organisms and refine pesticide formulations to use only the biologically effective enantiomer. This would lead to better-targeted pest control, lower environmental contamination, and reduced toxicity risks for non-target species.
EXAMPLES OF CHIRAL PESTICIDE MOLECULES AND THEIR BIOLOGICAL ACTIVITY
Now, let me share some interesting examples of pesticide molecules where stereochemistry plays a crucial role in their biological activity:
PERMETHRIN (Insecticide)
Pyrethroid
Permethrin is a synthetic insecticide belonging to the pyrethroid family. It has four stereoisomers due to the presence of two chiral centers in its cyclopropane ring, leading to different enantiomers and geometric isomers that influence its biological activity.
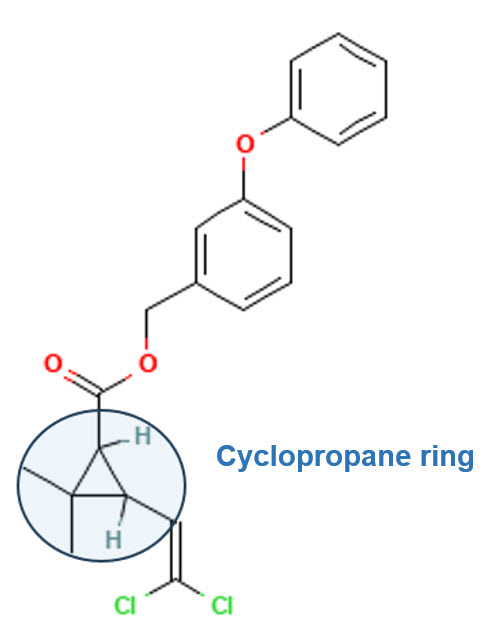
Chirality in Permethrin
Since permethrin has two chiral centers (C1 and C3 in the cyclopropane ring), the number of possible stereoisomers follows the rule:
Number of stereoisomers = 2n
where n = number of chiral centers.
Since permethrin has two chiral centers, we get:
22 = 4 stereoisomers
These four stereoisomers exist as two pairs of enantiomers, consisting of two trans enantiomers and two cis enantiomers.
The Four Stereoisomers of Permethrin:
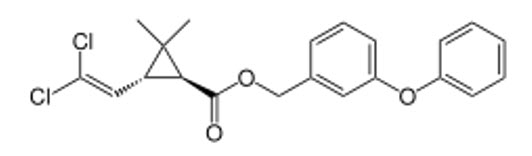 |
(1S,3R)-trans enantiomer |
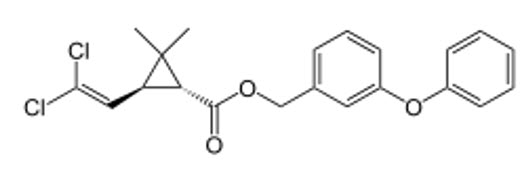 |
(1R,3S)-trans enantiomer |
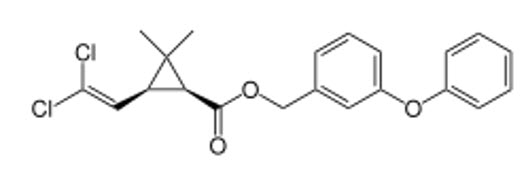 |
(1S,3S)-cis enantiomer |
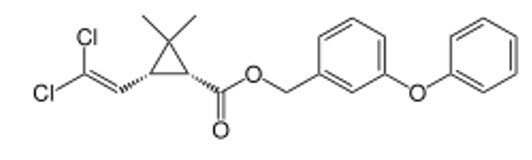 |
(1R,3R)-cis enantiomer |
Each enantiomeric pair consists of two molecules that are non-superimposable mirror images:
- (1R,3S)-trans-permethrin ↔ (1S,3R)-trans-permethrin (Enantiomers)
- (1R,3R)-cis-permethrin ↔ (1S,3S)-cis-permethrin (Enantiomers)
Chiral Centers in Permethrin:
The two chiral centers in permethrin are:
- C1 (Cyclopropane carbon)
- C3 (Cyclopropane carbon)
Each of these can exist in either the R or S configuration, leading to the four stereoisomers:
Isomer Name |
C1 Configuration |
C3 Configuration |
Type |
(1R,3S)-trans-permethrin |
R |
S |
Active |
(1S,3R)-trans-permethrin |
S |
R |
Active |
(1R,3R)-cis-permethrin |
R |
R |
Less active |
(1S,3S)-cis-permethrin |
S |
S |
Less active |
Geometric Isomerism: Trans vs. Cis Forms
Among the four stereoisomers, the trans-enantiomers exhibit strong insecticidal activity by targeting insect sodium channels, leading to paralysis and death.
The biologically active enantiomers are:
- (1R,3S)-trans-permethrin
- (1S,3R)-trans-permethrin
The cis-isomers (1R,3R and 1S,3S) are significantly less active but still present in commercial formulations.
Technical-Grade Permethrin: Composition of Enantiomers
Technical-grade permethrin is typically a mixture of all four stereoisomers.
Typical Enantiomer Composition in Technical Permethrin
Isomer |
Configuration |
Approximate % in Technical Permethrin |
Biological Activity |
(1R,3S)-trans-permethrin |
R, S |
25% |
Highly active |
(1S,3R)-trans-permethrin |
S, R |
25% |
Highly active |
(1R,3R)-cis-permethrin |
R, R |
25% |
Less active |
(1S,3S)-cis-permethrin |
S, S |
25% |
Less active |
Thus, technical permethrin is generally composed of:
- 50% trans-isomers (biologically active fraction)
- 50% cis-isomers (less active fraction)
Commercial Variants with Enriched Enantiomers
Some technical grades are enriched in the (1R)-enantiomers, which have stronger insecticidal activity.
"1R-trans-permethrin" refers to permethrin enriched in (1R,3S) and (1R,3R) forms, enhancing insecticidal properties.
Some high-performance technical grades contain ≥80% (1R)-enriched permethrin, improving efficiency and reducing environmental residues.
BIFENTHRIN (Insecticide)
Pyrethroid
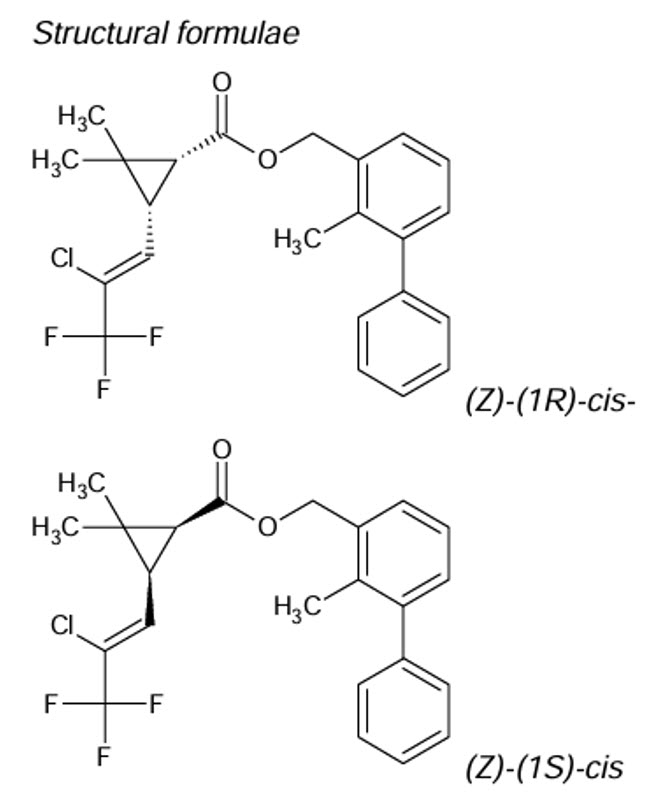
Tech. is >93% consisting of >97% cis- isomer and <3% trans- isomer. The cis isomer is the active form, the trans isomer shows very little insecticidal activity. Commercial formulations are typically enriched in the Cis- form.
DELTAMETHRIN (Insecticide)
Pyrethroid
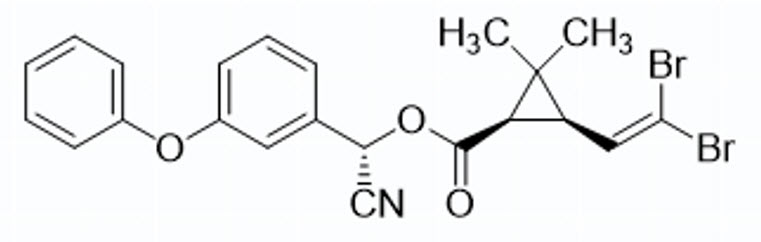
Only one of its 8 possible stereoisomers (the (1R,3R,αS) form) is highly active. The other stereoisomers show minimal insecticidal effects. This is why commercial deltamethrin is produced as a single isomer. Deltamethrin is the first pyrethroid composed of a single isomer of 8 stereoisomers selectively prepared.
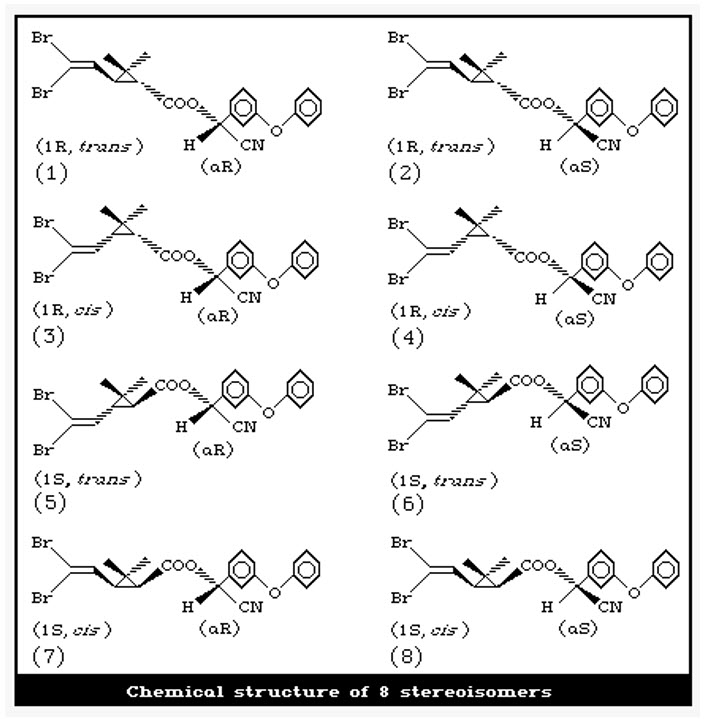
MECOPROP (Herbicide)
Mecoprop, or methylchlorophenoxypropionic acid (MCPP), is a common general use herbicide, a member of the chlorophenoxy herbicide group.
Mecoprop is a mixture of two enantiomers (non-superimposable mirror images of each other) with one active (R)-(+)- enantiomer possessing the herbicidal activity.
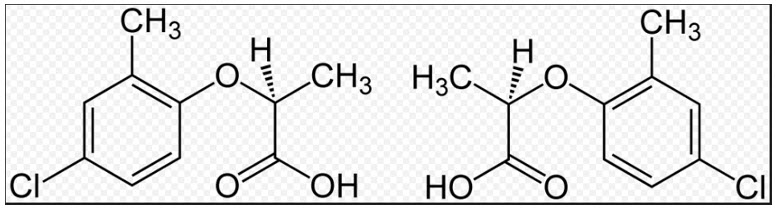
Structures of the two enantiomeric forms (S left, R right) of Mecoprop.
R-enantiomer: highly active against broadleaf weeds. S-enantiomer: virtually inactive.
Contains a chiral carbon with different groups creating asymmetry. Modern formulations use pure R-isomer (MECOPROP-P)
MYCLOBUTANIL (FUNGICIDE)
Triazole
The technical material is an isomeric equimolar mixture of the R- and S-forms.
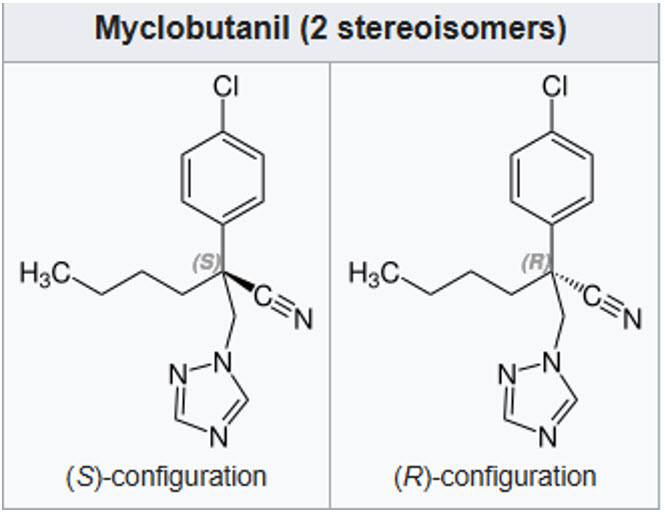
The R-enantiomer has strong antifungal properties, while the S-enantiomer exhibits minimal activity. Chiral center at carbon bearing the triazole group
INDOXACARB (INSECTICIDE)
Oxadiazine
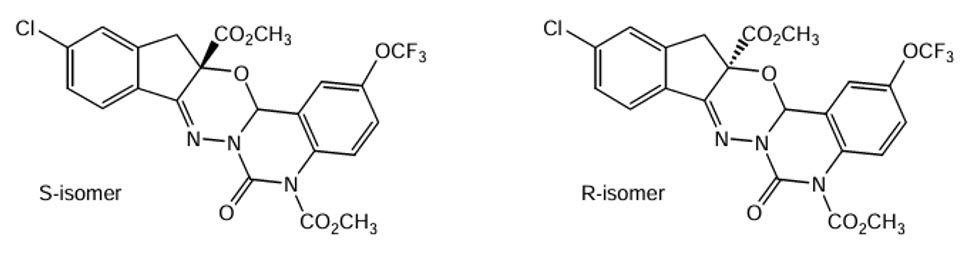
Indoxacarb is a racemic mix of R and S isomers (25:75). Only the S-isomer is insecticidally active. Indoxacarb has one chiral center. Two enantiomers are possible. The S isomer is the insecticidally active isomer, while the R isomer is insecticidally inactive. Chiral center involves sulfur-based stereochemistry.
METOLACHLOR AND S-METOLACLOR(HERBICIDE)
Chloroacetamide
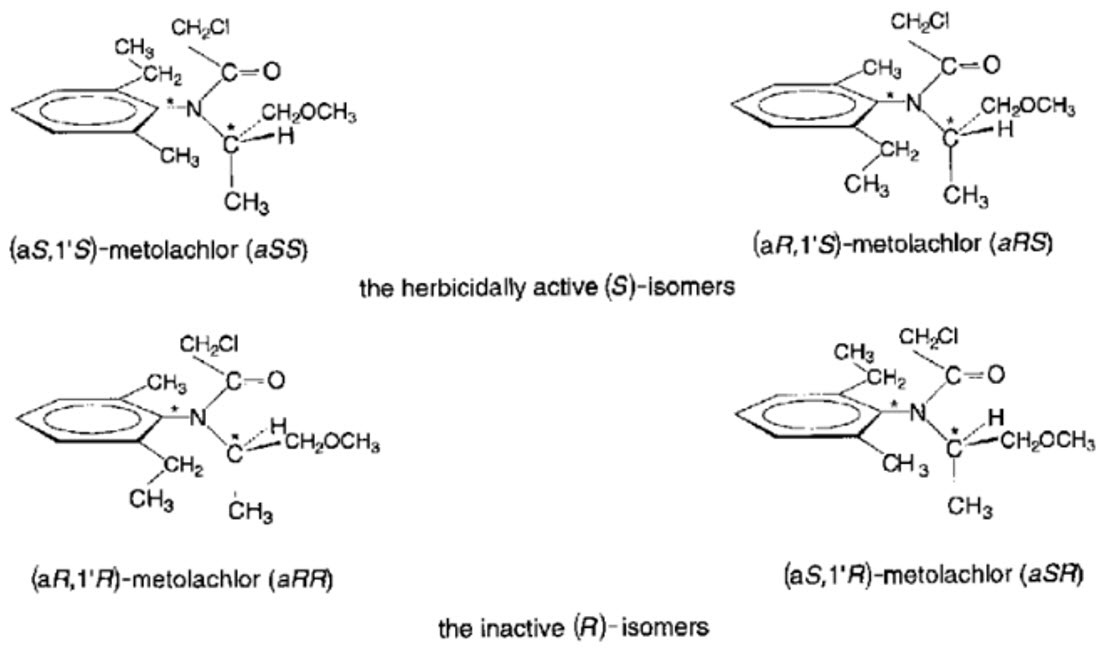
Metolachlor consists of four stable stereoisomers due to the presence of two chiral centers, resulting in diastereomers (non-mirror images), enantiomers (mirror images), and atropisomers (conformational isomers). While enantiomers share identical chemical and physical properties—except for the direction they rotate plane-polarized light—they can exhibit different interactions with chiral systems in the environment, affecting factors such as soil metabolism rates and degradation products. This difference explains why one enantiomer may demonstrate higher biological activity than its counterpart. In contrast, diastereomers and atropisomers possess distinct physical properties, such as solubility, melting point, and density, while their chemical properties remain similar but not identical.
Metolachlor is a racemic mixture comprising equal amounts of two R-enantiomers and two S-enantiomers.
S-Metolachlor is a refined product containing only the S-enantiomers, which make up 50% of the original racemic mixture that included both S- and R-enantiomers. These S-enantiomers demonstrate approximately 1.6 times greater herbicidal activity compared to the R-enantiomers. This enhanced effectiveness results from specific chiral interactions with target enzymes, which is why S-metolachlor has become the preferred active ingredient for weed.
FLUAZIFOP-BUTYL (HERBICIDE)
Aryloxyphenoxypropionate
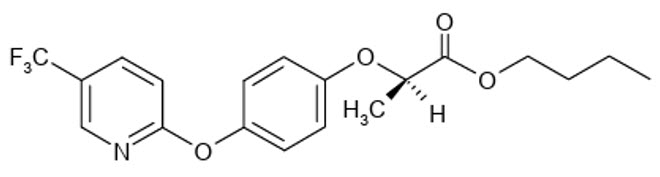
Racemate

Fluazefop-P-butyl, R-enantiomer

Fluazifop butyl, S-enantiomer
FLUAZIFOP-BUTYL is a chiral molecule that exists as R- and S-enantiomers, with only the R-isomer exhibiting significant herbicidal activity, while the S-isomer is essentially inactive. The commercial fluazifop-butyl formulation is a racemic mixture containing both enantiomers in a 50:50 w/w ratio, with its biological activity primarily attributed to the R-enantiomer. This enantiomer delivers the same level of herbicidal efficacy at half the application rate of the racemic mixture.
FLUAZIFOP-P-BUTYL is a refined commercial product containing the purified R-enantiomer with an enantiomeric purity of 96-99% R and only 1-4% S-enantiomer. This optimized formulation was developed to enhance efficiency by concentrating the active enantiomer responsible for the herbicidal effect.
TEBUCONAZOLE (FUNGICIDE)
Triazole
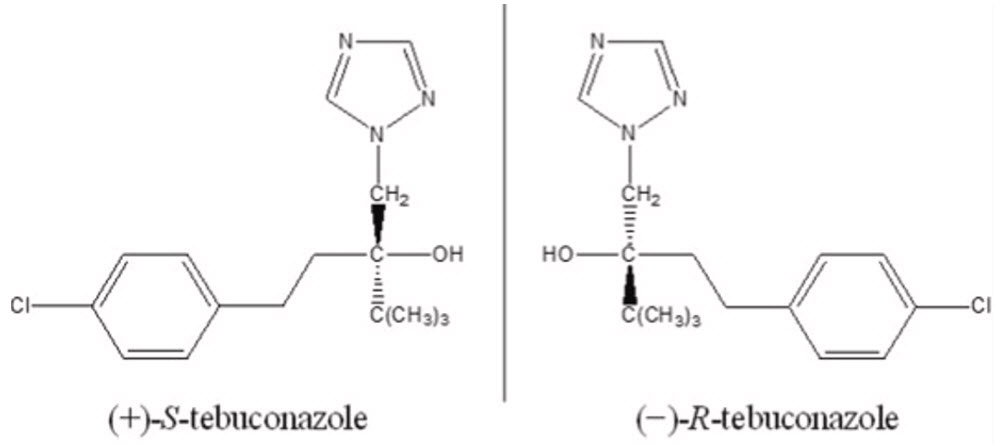
Tebuconazole molecule is chiral, with the technical material consisting of an isomeric mixture of (S)- and (R)-enantiomers. The (R)-enantiomer exhibits stronger fungicidal activity, while the (S)-enantiomer is significantly less effective. Commercial formulations typically contain the racemic mixture, which includes both enantiomers in equal proportions.
CYHALOTHRIN. LAMBDA-CYHALOTHRIN
Pyrethroid
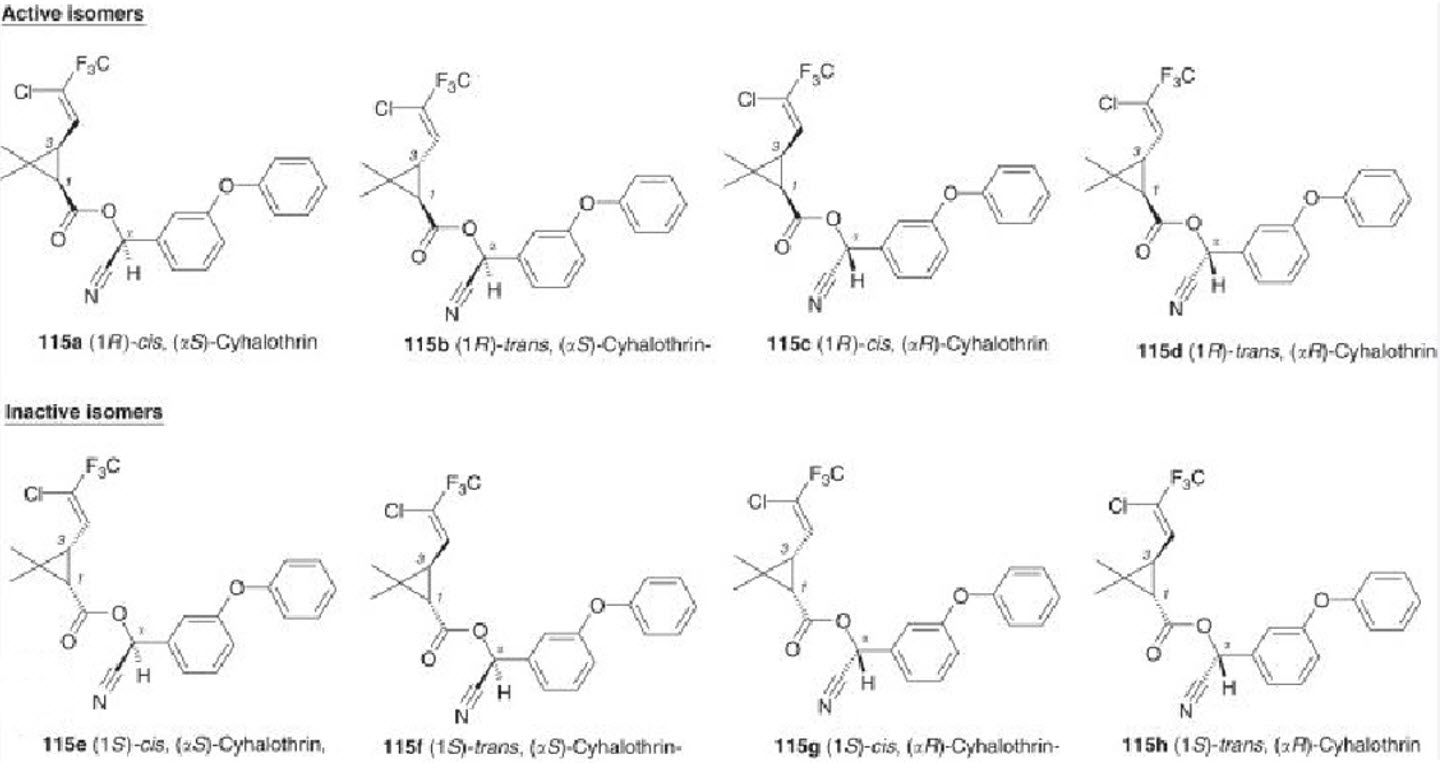
Cyhalothrin contains 8 stereoisomers. Cyhalothrin exhibits both geometric (cis/trans) and optical isomerism. Its biological activity is primarily associated with a specific combination of the cis arrangement and the R,S configuration. As a complex molecule with multiple types of isomerism, its efficacy depends on precise stereochemical composition.
Lambda-Cyhalothrin is a mixture of two isomers of the product, the R and S forms in the alpha carbon. 1:1 mixture of (S)-α-cyano-3-phenoxybenzyl-(Z)-(1R,3R)-3-(2-chloro-3,3,3trifluoroprop-1-enyl) -2,2-dimethylcyclopropane carboxylate and (R)-α-cyano3-phenoxybenzyl (Z)-(1S,3S)-3-(2-chloro-3,3,3-trifluoroprop-1-enyl)-2,2dimethylcyclopropane carboxylate.
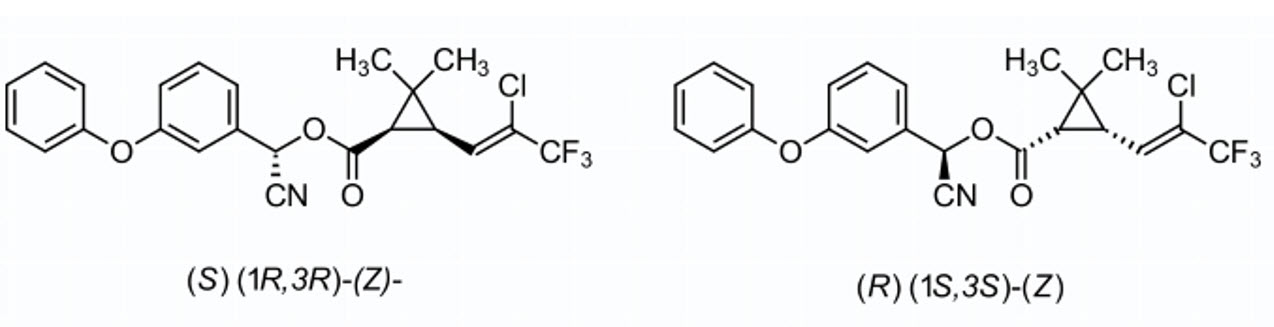
Although cyhalothrin and lambda-cyhalothrin are isomers, only lambda-cyhalothrin is supported by the manufacturer and therefore intended as a replacement for cyhalothrin.
PROPICONAZOLE (FUNGICIDE)
Triazole
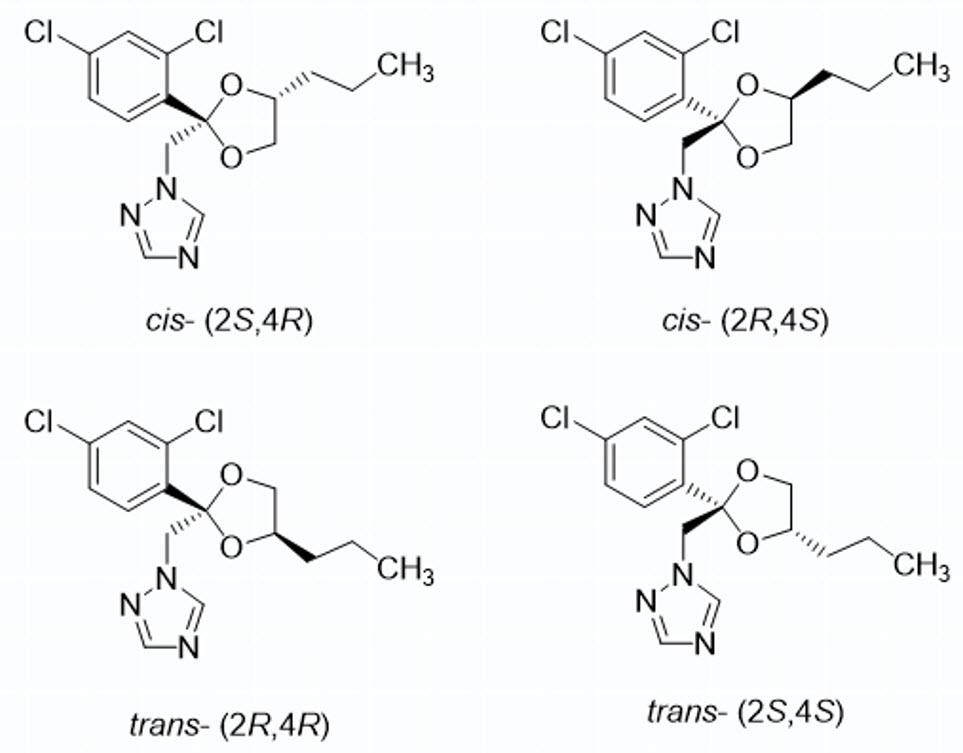
Propiconazole is a chiral molecule with two chiral centers, resulting in four stereoisomers that exist as cis- and trans-diastereomers. It is a racemic mixture in which all stereoisomers exhibit biological activity, though the (2R,4S) and (2S,4S) diastereomers demonstrate the highest fungicidal efficacy, while the other configurations have reduced effectiveness.
SPIROXAMINE (FUNGICIDE)
Spiroketalamine
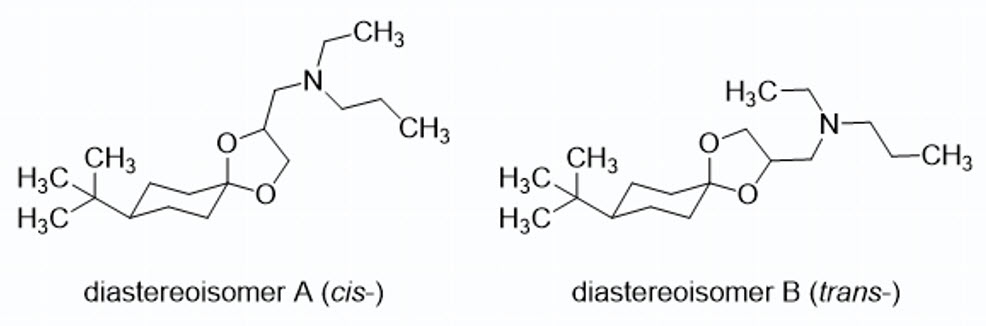
Spiroxamine is a fungicide composed of two diastereomers, A (cis) and B (trans), each existing as a pair of enantiomers (A1, A2, B1, and B2), resulting in a total of four stereoisomers. The diastereomers are present in a ratio of 50.7% (cis) to 44.3% (trans). Its multiple chiral centers contribute to complex stereochemistry, where the specific spatial arrangement is crucial for optimal fungicidal activity, and different isomers exhibit varying levels of potency.
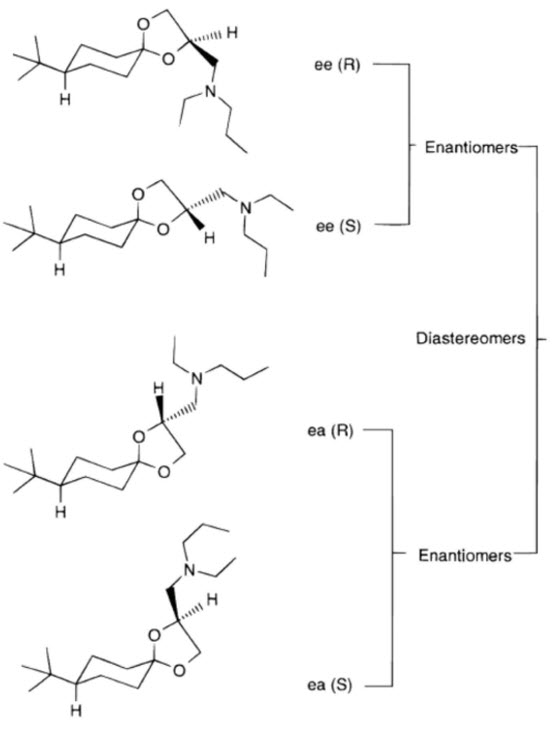
Diastereomers A1, A2, and B1, B2
EPOXICONAZOLE (FUNGICIDE)
Triazole

Epoxiconazole has three chiral centers creating multiple stereoisomers. (2R,3S)-isomer shows highest biological activity, other configurations have reduced effectiveness. Even though epoxiconazole is produced and commercialized as a racemic compound, recent publications indicate that the optically pure (2S,3R) enantiomer of epoxiconazole is much more active against pathogenic fungi than its (2R,3S)-enantiomer, whereas the plant growth regulatory activity mainly rests in the (2R,3S)-enantiomer.
PROTHIOCONAZOLE (FUNGICIDE)
Triazolinthione
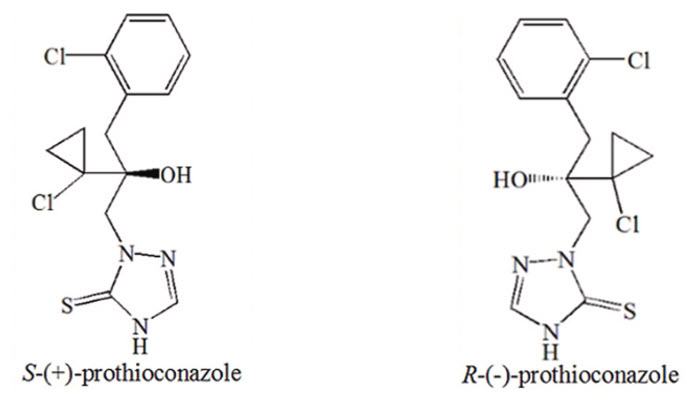
Prothioconazole is a chiral molecule with multiple possible stereoisomers, where its stereochemistry influences metabolism in plants and a specific configuration is required for optimal activity. The technical material is a racemate containing equal amounts of (R)- and (S)-Prothioconazole. The (R)-(-)-enantiomer exhibits significantly higher fungicidal activity, showing 9–18 times greater efficacy than the (S)-(+)-enantiomer.
Conclusion
Stereoisomerism is a fundamental factor influencing the biological activity, environmental fate, and toxicity of pesticide molecules. The presence of multiple stereoisomers within a single pesticide formulation can lead to significant variability in efficacy, with some isomers contributing little to pest control while others may pose ecological risks. Current pesticide formulations predominantly contain racemic mixtures, despite evidence that one enantiomer often exhibits superior activity. Advancing enantioselective pesticide development—where only the most effective stereoisomer is used—offers a promising path toward more efficient and environmentally responsible agrochemical solutions. Future research should focus on stereochemical optimization in pesticide design, regulatory frameworks supporting single-isomer formulations, and analytical techniques for assessing enantiomer-specific behavior. By prioritizing stereochemistry in pesticide development, the agricultural industry can enhance pest management while reducing environmental contamination and non-target toxicity.