Abstract
This review covers the role of adjuvants in the agrochemical industry, focusing on their functions, physicochemical mechanisms of action, and regulatory aspects. It also discusses the structures and properties of key adjuvants, as well as the shift toward sustainable solutions in global market trends.
INTRODUCTION TO ADJUVANTS IN AGROCHEMICALS
In modern agriculture, farmers and agronomists are always looking for ways to boost crop production while reducing environmental impact. One often overlooked tool in this effort is the use of adjuvants. These substances, added to pesticide formulations or spray mixtures, play a key role in enhancing the performance of herbicides, insecticides, and fungicides.
Adjuvants improve the effectiveness of agrochemicals in various ways, such as enhancing spray coverage, improving adhesion to plants, and increasing penetration through leaf surfaces. By making pesticides more efficient, adjuvants help reduce the amount needed, lowering both environmental impact and production costs. This supports the global shift toward more sustainable farming.
As we delve deeper into the world of adjuvants, we'll explore their types, mechanisms of action, and the role they play in modern agricultural practices. Understanding these essential additives is key to unlocking their full potential in our ongoing quest for more efficient, sustainable, and productive farming systems.
ADJUVANTS: FUNCTIONS AND TYPES
What Are Agricultural Adjuvants?
Definition: Agricultural adjuvants are substances added to herbicides, insecticides, fungicides, or other crop protection products to enhance their performance. While adjuvants are not used as standalone treatments, they play a crucial role in optimizing the effectiveness of active ingredients by improving factors such as coverage, penetration, and retention on target surfaces.
Function: Adjuvants cannot be applied independently, but they are integral to enhancing the activity and efficiency of other crop protection agents.
Types: Agricultural adjuvants modify the physicochemical properties of spray solutions to facilitate better interaction between the active ingredient and the target plant.
Agricultural adjuvants fall into 2 main categories depending upon their functional properties:
ADJUVANTS THAT ENHANCE THE EFFICACY OF THE PESTICIDE ACTIVE CONSTITUENT
ADJUVANTS THAT IMPROVE THE EASE OF APPLICATION OF THE PESTICDE TANK MIX
It is important to note that many adjuvants can fulfill multiple roles, simultaneously enhancing both pesticide efficacy and application efficiency. The following functional categories provide examples of how adjuvant products can be classified, though a single adjuvant may support multiple functions within these categories.
MECHANISMS OF ENHANCED EFFICACY BY ADJUVANTS
WETTERS/SPREADERS (enhance adhesion to and spreading of spray droplets on target surfaces by reducing the surface tension of the pesticide formulation and improving coverage).
This category may include:
NON-IONIC SURFACTANTS
ANIONIC SURFACTANTS
CATIONIC SURFACTANTS
AMPHOTERIC SURFACTANTS
ORGANO-SILICATE SURFACTANTS
ACIDIFIED SURFACTANTS
Physicochemical mechanism of action:
Surfactants (also called wetter-spreaders) are adjuvants that lower the surface tension of spray droplets. This allows gravity to collapse and flatten the spherical spray droplet to the leaf surface, which in turn allows droplets to spread over a larger area. Surfactants can also reduce the amount of bounce a droplet will experience when it hits a leaf, which allows for greater success at depositing.
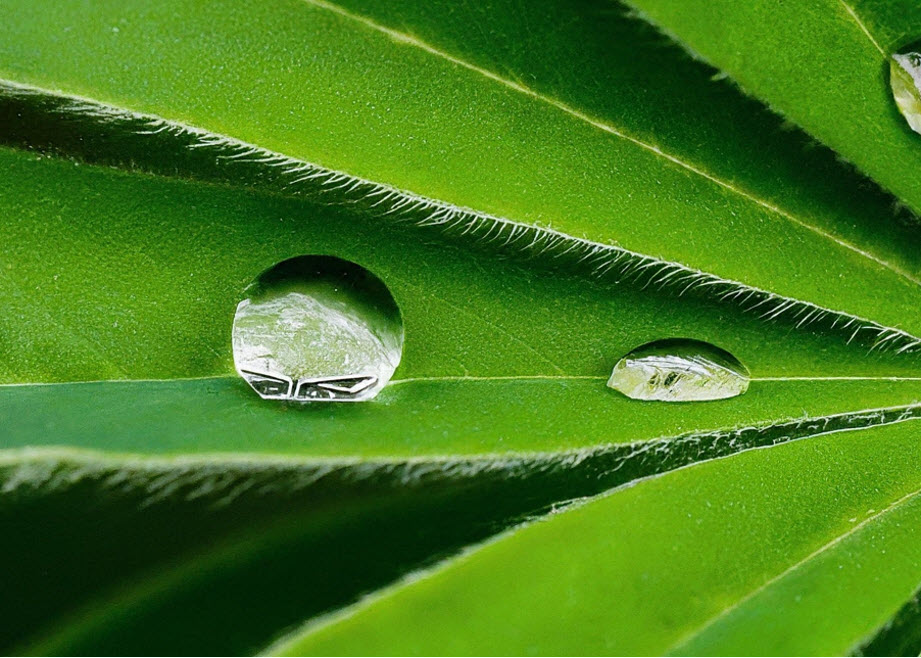
A droplet of the pesticide tank mix without spreader adjuvant (1),
and with spreader adjuvant (2)
STICKERS (Increase the adhesion of pesticides to target surfaces). This category of adjuvants may include:
LATEX-BASED
TERPENE/PINOLENE
PYRROLIDONE-BASED
Physicochemical mechanism of action:
Stickers are an important class of adjuvants in agrochemical applications, designed to enhance the adhesion of pesticides to target surfaces such as plant leaves. Let's explore the physicochemical mechanisms of action for the three types of stickers mentioned above: latex-based, terpene/pinolene, and pyrrolidone-based.
General Action Mechanism of Stickers:
Before exploring specific types, it's essential to understand the general role of stickers: they create a physical bond between the pesticide and the plant surface, helping to resist removal by rain, wind, or irrigation, and often altering the drying characteristics of spray droplets.
Now, let's examine each type in detail:
LATEX-BASED Stickers:
Latex-based stickers are typically made from synthetic polymer emulsions.
Physicochemical Mechanism:
FILM FORMATION:
As water evaporates from the spray droplet, latex particles coalesce to form a continuous film. This film physically encapsulates the pesticide molecules.
ADHESION:
The polymer chains in latex can interact with both the plant cuticle (through Van der Waals forces and hydrogen bonding) and the pesticide molecules. This creates a strong bridge between the pesticide and the plant surface.
ELASTICITY:
Latex films often retain some elasticity, allowing them to flex with the plant surface without cracking.
HYDROPHOBICITY:
Many latex formulations are designed to be hydrophobic once dried, providing water resistance.
TERPENE/PINOLENE-BASED Stickers:
These are derived from natural pine resins and consist of polyterpenoid compounds.
Physicochemical Mechanism:
RESIN FORMATION:
Terpene molecules polymerize as the carrier solvent evaporates, forming a resinous layer.
STRONG ADHESION:
The resinous nature provides excellent adhesion to waxy plant surfaces through hydrophobic interactions.
WATER RESISTANCE:
Terpene polymers are highly hydrophobic, creating a water-repellent barrier.
PLASTICITY:
The resin remains somewhat plastic, allowing it to expand and contract with the plant surface.
PYRROLIDONE-BASED STICKERS:
These adjuvants are typically based on polyvinylpyrrolidone (PVP) or related compounds.
Physicochemical Mechanism:
HYDROGEN BONDING:
PVP can form hydrogen bonds with both the plant surface and many pesticide molecules. This creates a strong, but reversible, adhesion.
FILM CHARACTERISTICS:
Forms a clear, flexible film upon drying. The film is often hygroscopic, which can help in maintaining pesticide solubility.
Comparative Aspects:
WEATHER RESISTANCE:
Terpene-Based stickers often provide the best rainfastness.
Latex-Based stickers can offer good UV resistance.
Pyrrolidone-Based stickers may be more susceptible to wash-off but can rehydrate.
DURATION OF EFFECT:
Terpene and Latex-Based stickers typically provide longer-lasting adhesion.
Pyrrolidone-Based stickers may offer shorter but more controlled release.
PLANT SURFACE INTERACTION:
Terpene-Based stickers work particularly well on waxy surfaces.
Latex and Pyrrolidone-Based stickers can be effective on a broader range of surface types.
ENVIRONMENTAL CONSIDERATIONS:
Terpene-Based stickers are often considered more environmentally friendly due to their natural origin.
Latex and Pyrrolidone-Based stickers may have longer environmental persistence.
Factors Affecting Performance:
PLANT SURFACE CHARACTERISTICS (WAXY, HAIRY, SMOOTH)
ENVIRONMENTAL CONDITIONS (TEMPERATURE, HUMIDITY, RAINFALL)
PESTICIDE FORMULATION COMPATIBILITY
APPLICATION METHOD AND DROPLET SIZE
In conclusion, stickers enhance pesticide efficacy by improving adhesion to plant surfaces through various physicochemical mechanisms. Latex-based stickers form flexible films, terpene/pinolene-based stickers create water-resistant resinous layers, and pyrrolidone-based stickers utilize hydrogen bonding for adhesion. The choice of sticker depends on the specific pesticide, target plant, environmental conditions, and desired duration of effect. Understanding these mechanisms allows for optimal selection and use of stickers in agrochemical applications, potentially leading to improved pest control with reduced environmental impact.
PENETRANTS (improve the transfer of active ingredients from the target surface to interior tissues). This category may include:
MINERAL OIL
VEGETABLE OIL
ESTERIFIED VEGETABLE OIL
ORGANO-SILICONE SURFACTANTS
ACIDIFIED SURFACTANTS.
Physicochemical mechanism of action:
Penetrants are essential adjuvants in agrochemical formulations, particularly for systemic pesticides that need to penetrate the leaf to be effective. These adjuvants are designed to enhance the movement of active ingredients from the plant surface into its internal tissues. Now, let's explore the physicochemical mechanisms of action for different types of penetrants:
General Mechanism of Penetrants:
Before diving into specific types, it's important to understand that penetrants generally work by: disrupting or modifying the plant cuticle structure; increasing the solubility of active ingredients in the cuticle; enhancing the diffusion of active ingredients through the cuticle and cell membranes.
Now, let's examine each type in detail:
MINERAL OIL Penetrants:
Physicochemical Mechanism:
CUTICLE DISRUPTION:
Mineral oils can partially dissolve or disorganize the waxy components of the plant cuticle. This creates temporary channels or pathways for pesticide movement.
CO-SOLVENT EFFECT:
Acts as a co-solvent for lipophilic pesticides, increasing their concentration in the cuticle.
SPREADING:
Reduces surface tension, allowing for better spreading of spray droplets.
PLASTICIZATION:
Can plasticize the cuticle, making it more permeable.
VEGETABLE OIL Penetrants:
Physicochemical Mechanism:
CUTICLE INTERACTION:
Like mineral oils, but often with reduced phytotoxicity. Can soften and partially solubilize cuticular waxes.
EMULSIFICATION:
Forms micro-emulsions with water and pesticide, enhancing pesticide availability.
MEMBRANE INTERACTION:
Some components may interact with cell membranes, temporarily increasing permeability.
ESTERIFIED VEGETABLE OIL Penetrants:
Physicochemical Mechanism:
ENHANCED LIPOPHILICITY:
Esterification increases the oil's affinity for the lipophilic cuticle. Improves penetration of both lipophilic and hydrophilic pesticides.
CUTICLE MODIFICATION:
More effective at disrupting the cuticle structure compared to non-esterified oils.
HUMECTANT PROPERTIES:
Some formulations can slow the drying of spray droplets, prolonging the absorption window.
ORGANO-SILICONE SURFACTANTS:
Physicochemical Mechanism:
In applications when a non-ionic surfactant improves penetration, the more effective approach is to match the pesticide’s polarity with a surfactant of similar polarity. When you closely match a pesticide and adjuvant, like dissolves like, and you will increase the rate of movement for specific pesticides into the leaf.
SUPER-SPREADING:
Dramatically reduces surface tension, allowing for extensive spreading on leaf surfaces. Increases contact area between the pesticide and leaf surface.
CUTICLE PENETRATION:
The small molecular size of silicone-based surfactants allows them to penetrate cuticle pores.
STOMATAL INFILTRATION:
Can facilitate the entry of spray solution through stomata, bypassing the cuticle barrier.
MOLECULAR ORIENTATION:
Forms oriented layers at interfaces, which can enhance pesticide diffusion.
ACIDIFIED SURFACTANTS:
Physicochemical Mechanism:
pH MODIFICATION:
Lowers the pH of the spray solution, which can be crucial for weak acid herbicides. Many herbicides are more readily absorbed in their non-ionized form at lower pH.
CUTICLE HYDRATION:
The acidic environment can increase cuticle hydration, enhancing permeability.
ION-TRAPPING EFFECT:
For weak acid herbicides, the pH gradient created can trap ions inside plant cells, enhancing accumulation.
MEMBRANE PERMEABILITY:
Slight acidification can affect cell membrane permeability, potentially enhancing pesticide uptake.
Comparative Aspects:
SPECIFICITY:
Organo-Silicate Surfactants often provide the most dramatic spreading.
Esterified Vegetable Oils typically offer the best rain fastness.
Acidified Surfactants are particularly effective with weak acid herbicides.
ENVIRONMENTAL CONSIDERATIONS:
Vegetable Oils and their Esters are often considered more environmentally friendly.
Mineral Oils may have longer environmental persistence.
PHYTOTOXICITY RISK:
Mineral Oils generally carry the highest risk of phytotoxicity.
Vegetable Oils and Organo-Silicates typically have lower phytotoxicity risks.
Factors Affecting Performance:
PLANT SPECIES AND GROWTH STAGE
ENVIRONMENTAL CONDITIONS (TEMPERATURE, HUMIDITY)
PESTICIDE PHYSICOCHEMICAL PROPERTIES
SPRAY VOLUME AND DROPLET SIZE
WATER QUALITY (HARDNESS, PH)
In summary, penetrants enhance pesticide efficacy by improving the transfer of active ingredients from the plant surface to interior tissues. They achieve this through various mechanisms including cuticle disruption, solubility enhancement, and modification of surface properties. The choice of penetrant depends on the specific pesticide, target plant, environmental conditions, and desired performance characteristics. Gaining insight into these physicochemical mechanisms enables the optimal selection and use of penetrants in agrochemical applications, potentially enhancing pest control while minimizing.
EXTENDERS (enhance the amount of time the active ingredient remains effective by increasing resistance to environmental degradation). This adjuvant category may include:
AMMONIUM SULPHATE
MENTHENE-BASED extenders
Physicochemical mechanism of action:
The mechanism of action of extender adjuvants in agrochemicals can be illustrated by considering two examples: ammonium sulfate and menthene-based extenders.
AMMONIUM SULPHATE (AMS) EXTENDERS:
Ammonium sulphate works as an extender through several mechanisms:
WATER CONDITIONING:
AMS acts as a water conditioner by binding to and neutralizing antagonistic cations like calcium, magnesium, and iron in hard water. This prevents these cations from binding to and deactivating the active ingredients in herbicides, particularly glyphosate.
pH MODIFICATION:
AMS slightly lowers the pH of the spray solution, which can enhance the solubility and absorption of weak acid herbicides.
IMPROVED UPTAKE:
The ammonium ions (NH4+) in AMS can enhance the absorption of certain herbicides through plant cuticles and cell membranes. This is particularly effective for herbicides that are weak acids, as they can form a more lipophilic ion pair with the ammonium, facilitating easier penetration into plant tissues.
REDUCED CRYSTALLIZATION:
By binding to cations, AMS helps prevent the formation of insoluble salts on leaf surfaces, which could otherwise reduce the effectiveness of the herbicide.
MENTHENE-BASED Extenders:
Menthene-based extenders, also known as pinolene or terpene polymers, work through different mechanisms:
FILM FORMATION:
These compounds form a physical film or coating over the spray droplets once they've been deposited on plant surfaces. This film is water-insoluble but permeable to the active ingredient.
EVAPORATION REDUCTION:
The film slows down the evaporation of water from spray droplets, keeping the active ingredient in a dissolved state for a longer period. This extends the time available for the active ingredient to penetrate the plant tissues.
WASH-OFF RESISTANCE:
The hydrophobic nature of the menthene-based film increases the rainfastness of the applied agrochemical. It helps the active ingredient resist being washed off by rain or irrigation.
UV PROTECTION:
Some menthene-based extenders can provide a degree of UV protection to light-sensitive active ingredients, slowing down their photodegradation.
Re-WETTING PROPERTIES:
In some formulations, these extenders can allow for re-wetting of the spray deposit under high humidity conditions, potentially extending the absorption window.
In both cases, the primary goal of these extender adjuvants is to prolong the effective lifespan of the active ingredient on the plant surface. This is achieved by either protecting it from environmental factors (like in the case of menthene-based extenders) or by enhancing its solubility and uptake (as with ammonium sulphate). The result is improved efficacy of the agrochemical, potentially allowing for reduced application rates or less frequent applications.
HUMECTANTS (increase the drying time of an aqueous spray deposit).These may include:
GLYCEROL
PROPYLENE GLYCOL
DIETHYL GLYCOL
POLYETHYLENE GLYCOL
SORBITOL
Physicochemical mechanism of action:
Humectants are adjuvants, either synthetic or naturally derived, that attract moisture from the environment or slow water evaporation, helping to retain moisture on plant leaves or soil surfaces. This enables humectants to enhance the foliar uptake of certain pesticides.
The longer the spray droplet stays in its liquid form, the more time certain pesticides will have to penetrate the leaf surface. This is particularly true of highly polar and water-soluble pesticides in situations when solubility is critical for the pesticide to be available to move into the plant. Once a polar pesticide dries on the surface (crystalizes), it no longer diffuses as rapidly into the leaf. For pesticide products that are not rapidly absorbed, prolonging the spray droplet’s drying time can be critical.
Humectants are substances that can absorb and retain moisture from the atmosphere. When used in agrochemical adjuvants, they can significantly influence the efficacy and persistence of the active ingredient.
Some humectants can soften the plant cuticle, making it easier for the active ingredient to penetrate the plant tissue. This can improve the efficacy of systemic herbicides and insecticides. Humectants can protect plants from environmental stress by slowing down the loss of moisture through their leaves. This is especially helpful in dry areas, where water is scarce, and plants need to hold onto as much moisture as possible to stay healthy.
MECHANISMS OF IMPROVED EASE OF APPLICATION BY ADJUVANTS
ACIDIFYING/BUFFERING AGENTS (adjust the pH of alkaline or acidic water and minimize decomposition of the pesticide through alkaline hydrolysis).
Physicochemical mechanism of action:
Acidifying/buffering agents are substances that can alter the pH of a solution. When used in agrochemical adjuvants, they can significantly influence the efficacy and stability of the active ingredient. Here are the primary physicochemical mechanisms through which these agents operate:
COMPATIBILITY ENHANCEMENT:
PREVENTING PRECIPITATION:
Acidifying/buffering agents can help to prevent the precipitation of certain active ingredients or other components of the spray solution in the tank mix, ensuring better compatibility and stability.
REDUCED ENVIRONMENTAL IMPACT:
HYDROLYSIS CONTROL:
By adjusting the pH, acidifying/buffering agents can influence the rate of hydrolysis of certain active ingredients, potentially reducing their environmental impact.
Commonly used acidifying/buffering agents in agrochemical adjuvants include:
PHOSPHORIC ACID
CITRIC ACID
ACETIC ACID
SODIUM HYDROXIDE
POTASSIUM HYDROXIDE
ETHYLENEDIAMINETETRAACETIC ACID (EDTA) AND ITS DERIVATIVES
ANTI-FOAMING/DE-FOAMING AGENTS (reduce or suppress the formation of foam in the spray tank, preventing foam overflow). This adjuvant category may include:
SILICONE-BASED AGENTS (POLYDIMETHYLSILOXANE)
OIL-BASED AGENTS
PARTICLE-BASED AGENTS
FATTY ALCOHOLS AND ESTERS
Physicochemical mechanism of action:
Anti-foaming or de-foaming agents are important adjuvants in agrochemical formulations. They help prevent or break down foam that can form during the mixing and application of pesticides, herbicides, and other agricultural chemicals. Let's delve into the physicochemical mechanisms of action for these agents:
Foam Formation Basics:
Before discussing anti-foaming mechanisms, it's important to understand how foam forms: Foam consists of gas bubbles dispersed in a liquid phase. The foam structure is supported by thin liquid films called lamellae, which separate the gas bubbles. At the junctions where three or more bubbles meet, Plateau borders are formed, which help redistribute liquid from the thin films. The thin film region or liquid phase is where most of the liquid resides, while the gas phase fills the bubbles themselves. The interface, also known as the 2-D surface phase, is where surfactants accumulate, reducing surface tension and stabilizing the foam. Surfactants in agrochemical formulations, or large polymeric molecules, can enhance foam stability by reinforcing these thin films and interfaces, preventing the collapse of the foam structure.
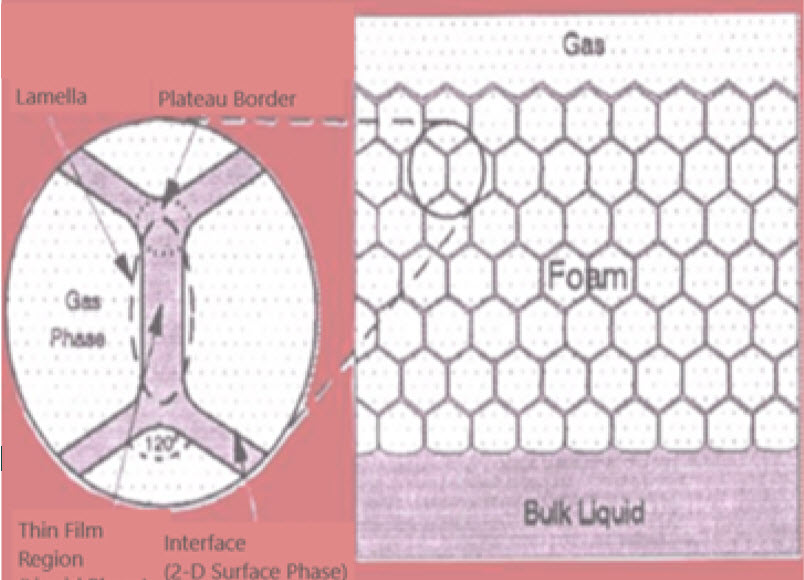
Mechanisms of Anti-foaming/De-foaming Agents:
SPREADING MECHANISM:
Many anti-foaming agents are insoluble oils or hydrophobic particles. These agents spread rapidly on the surface of foam bubbles. As they spread, they displace the foam-stabilizing surfactants. This destabilizes the bubble film, causing it to rupture.
BRIDGING-DEWETTING MECHANISM:
Some anti-foaming particles can bridge across the thin liquid films between bubbles. As these particles de-wet (liquid recedes from their surface), they create weak points in the foam structure. These weak points become sites for bubble rupture and foam collapse.
ENTERING-SPREADING-BRIDGING MECHANISM:
This is a combination of the above two mechanisms. The anti-foaming agent enters the foam lamella (thin liquid film between bubbles). It then spreads within this film, creating a lens. The lens bridges the two sides of the lamella, leading to film rupture.
MODIFICATION OF SURFACE PROPERTIES:
Some anti-foaming agents work by altering the surface properties of the liquid. They can increase surface tension, making it harder for bubbles to form and persist. They may also increase the drainage rate of liquid from foam films, destabilizing the foam structure.
Common types of anti-foaming/de-foaming agents:
SILICONE-BASED AGENTS (POLYDIMETHYLSILOXANE):
Often used are polydimethylsiloxane (PDMS) oils or silicone emulsions. These are highly effective due to their low surface tension and insolubility in water. They spread rapidly on foam surfaces, displacing foam-stabilizing surfactants.
OIL-BASED AGENTS:
Mineral oils or vegetable oils are used. They work similarly to silicone oils but are generally less effective.
PARTICLE-BASED AGENTS:
Hydrophobic silica or wax particles are common. These work primarily through the bridging-de-wetting mechanism. They're used in combination with oils for enhanced effectiveness.
FATTY ALCOHOLS AND ESTERS:
These disrupt foam by altering surface properties and interfering with surfactant arrangements at interfaces.
Factors Affecting Anti-foaming Efficiency:
PARTICLE SIZE AND HYDROPHOBICITY (FOR PARTICLE-BASED AGENTS)
SPREADING COEFFICIENT (FOR OIL-BASED AGENTS)
COMPATIBILITY WITH THE FORMULATION INGREDIENTS
TEMPERATURE (CAN AFFECT SPREADING AND SOLUBILITY)
pH OF THE SYSTEM
To sum up, anti-foaming/de-foaming agents work primarily by destabilizing foam structures through various physicochemical mechanisms. Their action helps ensure proper mixing, accurate dosing, and efficient application of agrochemicals, ultimately contributing to the overall effectiveness of the treatment.
COMPATIBILITY AGENTS (permit the mixing of different agrochemicals by preventing antagonism between different ingredients in the spray solution).
One example of this category is ammonium sulfate (AMS), which stands out among compatibility agents for its outstanding role in enhancing pesticide performance by effectively mitigating hard water effects and maximizing the efficacy of active ingredients in tank mixes.
AMMONIUM SULPHATE
Physicochemical mechanism of action:
Compatibility agents play important role in modern agriculture, allowing farmers and applicators to mix different agrochemicals in a single tank, saving time, labor, and resources. Ammonium sulphate (AMS) is a particularly versatile compound that serves multiple functions in agrochemical applications, including as a compatibility agent. Let's explore the physicochemical mechanisms of action for ammonium sulphate as a compatibility agent:
General Role of Compatibility Agents:
Before diving into the specific mechanisms of AMS, it's important to understand the general function of compatibility agents:
They prevent adverse interactions between different agrochemicals in a spray solution, maintain the stability and efficacy of mixed formulations and can enhance the overall performance of the spray mixture.
Ammonium Sulphate (AMS) as a Compatibility Agent:
Chemical Formula: (NH₄)₂SO₄
Physicochemical Mechanisms of Action:
ION SEQUESTRATION:
AMS acts as a complexing agent, binding to problematic metal ions in the spray solution. The primary purpose of AMS in agricultural sprays is to mitigate the negative effects of hard water cations like calcium (Ca²⁺), magnesium (Mg²⁺), and iron (Fe²⁺) that can interfere with the efficacy of certain herbicides, particularly glyphosate. This prevents these ions from interacting with and potentially deactivating certain pesticides.
pH BUFFERING:
AMS has a slight acidifying effect on the spray solution. It can help maintain a stable pH, which is crucial for the stability and efficacy of many pesticides.
SALT FORMATION:
The sulphate ion (SO₄²⁻) can form salts with various cations in the spray solution. This can prevent these cations from forming insoluble complexes with certain pesticides.
SOLUBILITY ENHANCEMENT:
For some pesticides, the presence of ammonium ions can enhance their solubility. This is particularly important for weak acid herbicides like glyphosate.
PREVENTION OF ANTAGONISM:
AMS can prevent antagonistic interactions between different pesticides and hard water. For example, it can mitigate the negative effects of hard water cations on herbicide efficacy.
Specific Interactions and Benefits:
GLYPHOSATE COMPATIBILITY:
AMS is particularly beneficial when mixing glyphosate with other herbicides. It prevents the formation of glyphosate-metal complexes that can reduce efficacy.
COMPATIBILITY WITH PHENOXY HERBICIDES:
AMS can enhance the compatibility and efficacy of mixtures containing phenoxy herbicides (e.g., 2,4-D) with other agrochemicals.
MICRONUTRIENT MIXTURES:
When mixing pesticides with micronutrient solutions, AMS can help prevent precipitation and maintain solution stability.
SUSPENSION CONCENTRATE (SC) FORMULATIONS:
AMS can help maintain the stability of SC formulations when mixed with other products.
Concentration Effects:
The amount of AMS needed depends on water quality, the specific agrochemicals being mixed, and their concentrations. Typical rates range from 8.5 to 17 pounds of AMS per 100 gallons of spray solution (1-2% w/v). Higher rates may be needed for extremely hard water or complex mixtures.
Factors Affecting Performance:
WATER QUALITY:
The hardness and pH of the water used for mixing significantly impact the effectiveness of AMS.
TEMPERATURE:
Solution temperature can affect the solubility and reactivity of various components.
ORDER OF ADDITION:
The sequence in which products are added to the tank can influence compatibility. AMS is typically added early in the mixing process.
FORMULATION TYPES:
The types of formulations being mixed (e.g., EC, WP, SC) can influence compatibility challenges.
Limitations and Considerations:
NOT UNIVERSAL:
While AMS is effective with many agrochemical mixtures, it's not a universal compatibility agent. Some combinations may require different or additional compatibility agents.
POTENTIAL FOR ANTAGONISM:
In rare cases, AMS itself can antagonize certain pesticides or tank mix components.
EQUIPMENT CONSIDERATIONS:
High concentrations of AMS can be corrosive to certain metals used in spray equipment.
ENVIRONMENTAL IMPACT:
AMS adds nitrogen to the system, which could be a consideration in sensitive ecosystems or nitrogen-restricted areas.
To conclude, ammonium sulphate serves as an effective compatibility agent in agrochemical mixtures through various physicochemical mechanisms. By sequestering ions, buffering pH, enhancing solubility, and preventing antagonistic interactions, AMS helps maintain the stability and efficacy of complex tank mixes. This allows for the simultaneous application of multiple agrochemicals, potentially improving pest control efficiency and reducing the number of required applications. However, its use should be carefully considered based on the specific agrochemicals being mixed, water quality, and environmental factors to ensure optimal performance and minimize potential negative impacts.
DRIFT-REDUCING AGENTS (alter the viscoelastic properties of the spray solution, yielding a coarser spray with greater DV0.1 and DV0.5 droplet sizes):
POLYACRYLAMIDES
POLYSACCHARIDES
Physicochemical mechanism of action:
Drift-reducing agents (DRAs) are crucial adjuvants in agrochemical applications, designed to minimize off-target movement of spray droplets. The two types - polyacrylamides and polysaccharides - work through similar physicochemical mechanisms to achieve this goal. Let's explore how these agents function:
General Mechanism of Drift-Reducing Agents:
The primary function of DRAs is to alter the viscoelastic properties of the spray solution, resulting in:
INCREASED DROPLET SIZE
REDUCED PROPORTION OF FINE DROPLETS
IMPROVED DROPLET VELOCITY
ENHANCED SPRAY PATTERN UNIFORMITY
These effects are achieved by modifying the fluid dynamics during the atomization process.
POLYCRYLAMIDES as drfit-reducing agents:
Polyacrylamides (PAMs) are synthetic, water-soluble polymers with high molecular weight.
VISCOELASTIC PROPERTIES:
PAMs increase the extensional viscosity of the spray solution. This resistive force opposes the breakup of liquid filaments during atomization.
During spray formation, PAM molecules become stretched and aligned in the direction of fluid flow. This molecular alignment creates resistance to further stretching and breakup of fluid elements. The result is the formation of larger droplets and fewer fine, drift-prone droplets.
MOLECULAR INTERACTIONS:
PAMs form hydrogen bonds with water molecules. These interactions create a network structure in the solution, further increasing its resistance to breakup.
Concentration Effects:
The effectiveness of PAMs is highly concentration dependent. At low concentrations, they can decrease droplet size due to reduced surface tension. Optimal drift reduction occurs at higher concentrations where viscoelastic effects dominate.
POLYSACCHARIDES as drift-reducing agents:
Polysaccharides used as DRAs are typically derived from natural sources like guar gum or xanthan gum.
SOLUTION PROPERTIES:
Polysaccharides form highly viscous solutions even at low concentrations. They exhibit non-Newtonian, shear-thinning behavior.
Like PAMs, polysaccharides increase the extensional viscosity of the spray solution. Their long, branched molecular structure resists elongational flow during atomization.
MOLECULAR INTERACTIONS:
Polysaccharides form extensive hydrogen bonding networks in aqueous solutions. This results in a gel-like structure that resists rapid deformation and breakup.
SHEAR-THINNING BEHAVIOR:
Under high shear (e.g., in the nozzle), viscosity decreases, allowing for normal flow. As droplets form and shear decreases, viscosity rapidly increases, stabilizing larger droplets.
Physicochemical Effects on Spray Characteristics:
Both polyacrylamides and polysaccharides affect key spray characteristics:
DROPLET SIZE DISTRIBUTION:
Increase in DV0.1 (droplet size where 10% of the spray volume is in smaller droplets). Increase in DV0.5 (volume median diameter). Reduction in the proportion of droplets smaller than 100-150 μm (those most prone to drift)
SPRAY PATTERN:
Generally, maintain or slightly narrow the spray angle. Can lead to a more uniform distribution of spray across the target area
DROPLET VELOCITY:
Larger droplets have higher kinetic energy and are less affected by wind.
Factors affecting performance:
MOLECULAR WEIGHT OF THE POLYMER
CONCENTRATION IN THE SPRAY SOLUTION
NOZZLE TYPE AND SPRAY PRESSURE
ENVIRONMENTAL CONDITIONS (TEMPERATURE, HUMIDITY)
INTERACTIONS WITH OTHER FORMULATION COMPONENTS
Considerations in Agrochemical Use:
COMPATIBILITY WITH ACTIVE INGREDIENTS AND OTHER ADJUVANTS
POTENTIAL FOR NOZZLE CLOGGING AT HIGH CONCENTRATIONS
IMPACT ON SPRAY COVERAGE AND EFFICACY
ENVIRONMENTAL FATE OF THE POLYMERS
In closing, polyacrylamides and polysaccharides function as drift-reducing agents by altering the viscoelastic properties of spray solutions. They increase extensional viscosity and resist fluid breakup during atomization, resulting in larger droplets less prone to drift. This physicochemical mechanism allows for more precise application of agrochemicals, reducing environmental impact and improving overall efficacy.
DYES (commonly used for spot- or boom-spraying herbicides to detect missed spots or avoid spraying a plant or area twice).
Physicochemical mechanism of action:
Dyes are an important category of adjuvants used in agrochemical applications, particularly in herbicide spraying operations. Their primary purpose is to enhance the visibility of the spray application, which serves several crucial functions. Let's explore the use of dyes in more detail:
Primary functions of dyes in agrochemical applications:
VISUAL MARKER:
Dyes provide a clear visual indication of where the herbicide has been applied. This is especially useful for spot treatments or when using boom sprayers over large areas.
APPLICATION ACCURACY:
Help operators avoid missing spots or overlapping spray patterns, ensuring more uniform coverage. Particularly valuable in situations where the herbicide effect is not immediately visible.
OVERSPRAY PREVENTION:
Assist in preventing accidental spraying of non-target plants or areas. Useful in precision applications near sensitive crops or in landscaped areas.
Types of Dyes Used:
WATER-SOLUBLE DYES:
Most used due to easy mixing with water-based herbicide formulations. Examples include fluorescent dyes like Rhodamine B or food-grade colorants.
OIL-SOLUBLE DYES:
Used in oil-based herbicide formulations or when an oil-based carrier is employed.
TEMPORARY MARKING DYES:
Designed to break down quickly (usually within a few days) under sunlight and weather conditions.
FOAM MARKING DYES:
Used in foam marking systems for field edge marking in large-scale applications.
Application Methods:
SPOT SPRAYING:
Dyes are particularly useful when treating individual weeds or small patches. The colored spray helps ensure complete coverage of the target plant.
BOOM SPRAYING:
In large-scale applications, dyes help operators maintain straight lines and avoid gaps or overlaps between passes. Useful for maintaining application records and verifying coverage patterns.
BACKPACK SPRAYERS:
Dyes help users track their progress when treating smaller areas or hard-to-reach spots.
Concentration and Visibility:
The concentration of dye used depends on the desired visibility and duration. Higher concentrations provide more intense coloration but may stain equipment or persist longer in the environment. Some dyes are formulated to be highly visible initially but fade quickly.
Environmental and Safety Considerations:
BIODEGRADABILITY:
Many modern dyes are designed to be biodegradable and environmentally friendly. They break down under sunlight and microbial action, minimizing long-term environmental impact.
NON-TOXICITY:
Dyes used in agriculture are typically non-toxic to plants, animals, and humans. Some are food-grade colorants, further ensuring safety.
STAINING POTENTIAL:
Users should be aware of the potential for staining clothing, skin, or equipment. Proper protective equipment and cleaning procedures are important.
Advanced Uses:
SPRAY PATTERN ANALYSIS:
Dyes can be used to analyze spray patterns and droplet distribution on specialized cards or surfaces. This helps in optimizing nozzle selection and spray techniques.
DRIFT ASSESSMENT:
In research or regulatory contexts, dyes can help visualize and quantify spray drift.
TRAINING AND DEMONSTRATION:
Colored sprays are valuable for training new operators or demonstrating application techniques.
Limitations and Considerations:
WEATHER IMPACT:
Heavy dew or rain can wash away the dye, reducing its effectiveness as a marker.
SOIL STAINING:
Some dyes may temporarily stain soil, which could be a concern in certain settings (e.g., ornamental gardens).
COMPATIBILITY:
Not all dyes are compatible with all herbicide formulations. It's important to check for any adverse interactions.
COST:
While generally inexpensive, the additional cost of dyes needs to be considered in large-scale operations.
In conclusion, dyes play an important role in enhancing the precision and efficiency of herbicide applications. By providing a visual indicator of spray coverage, they help reduce waste, minimize environmental impact, and improve the overall effectiveness of weed control efforts. Their use is particularly valuable in situations requiring high accuracy or when working with invisible or slow-acting herbicides. As with any agrochemical adjuvant, proper selection and use of dyes should consider the specific application needs, environmental conditions, and safety requirements.
EXAMPLES OF SPECIFIC ADJUVANTS AND THEIR CHEMICAL STRUCTURES
Here are some specific chemical structures of common adjuvant molecules along with product examples:
Trade Name |
Manufacturer |
Chemical Structure |
Chemical Formula |
Function |
Agral 90 |
Syngenta |
Nonylphenoxy polyethoxy ethanol. |
C₉H₁₉–C₆H₄–O–(CH₂CH₂O)ₙ–CH₂CH₂OH |
Wetting agent, spreader |
Silwet L-77 |
Momentive |
modified trisiloxane polyethoxylate |
(CH₃)₃SiO[Si(CH₃)(OCH₂CH₂)xO]nSi(CH₃)₃ |
Super spreader, wetting agent |
Break-Thru S240 |
Evonik Industries |
Polyether Trisiloxane surfactant |
C₆H₁₈O₆Si₃ |
Super spreader, penetrant, wetting agent |
Destiny HC |
WinField United |
Methylated Soybean Oils (MSOs) |
RCOOCH₃ |
Penetrant, herbicide enhancer |
LI-700 |
Loveland Products |
MSO concenttrate with Lecithin (phospholipids) |
RCOOCH₃ and C₅H₁₄NO₄P(C₁₇H₃₅COO)(C₁₇H₃₃COO) |
Drift reduction, acidifier, penetra |
Foamaster |
BASF |
Polydimethylsiloxane (PDMS), and Silica and Oil blend |
(CH₃)₃SiO[Si(CH₃)₂O]nSi(CH₃)₃ |
Antifoaming agent |
Bond |
Loveland Products |
Synthetic PVA latex, Alcohol Ethoxylate |
(C₄H₆O₂)n , and R-O-(CH₂CH |
Spreader sticker , Deposition aid , Rainfastness enhancer |
Penetrator Plus |
Helena Agri-Enterprises |
blend of light to mid-range petroleum oil and nonionic emulsifiers |
C12-C20 |
Penetrant, systemic herbicide enhancer |
This table covers various adjuvants that serve functions ranging from surfactants, penetrants, spreaders, and antifoaming agents to emulsifiers and stickers. Each adjuvant is optimized to enhance the effectiveness of agrochemicals under different conditions and formulations.
ADJUVANTS AND MAIN CROPS: KEY RECOMMENDATIONS
CEREAL CROPS:
WHEAT, RICE, MAIZE (CORN), BARLEY, OATS, RYE, SORGHUM
FRUIT CROPS:
APPLES, ORANGES, BANANAS, GRAPES, MANGOES, BERRIES (e.g., STRAWBERRIES, BLUEBERRIES)
VEGETABLE CROPS:
TOMATOES, CARROTS, CABBAGES, LEAFY GREENS (e.g., SPINACH, KALE), BELL PEPPERS
ROOT AND TUBER CROPS:
POTATOES, CASSAVA (YUCA), YAMS
OIL CROPS:
SOYBEANS, OIL PALM, COCONUTS, SUNFLOWERS.
Examples of recommended adjuvant types for some crops
CORN (MAIZE):
Consider using nonionic surfactants (NIS) or crop oil concentrates. These enhance pesticide coverage and penetration.
SOYBEANS:
Nonionic surfactants (NIS) or methylated seed oils (MSO) improve pesticide efficacy.
WHEAT:
NIS or crop oil concentrates.
COTTON:
NIS or MSO are commonly used with herbicides.
RICE:
NIS or MSO work well with rice herbicides.
FRUITS (e.g., APPLES, GRAPES):
NIS or organosilicon-based surfactants are suitable for improving pesticide coverage.
VEGETABLES (e.g., TOMATOES, PEPPERS):
NIS or crop oil concentrates are commonly used.
REGULATION OF ADJUVANTS IN THE US
In the United States, the regulatory landscape for agrochemical adjuvants is complex and somewhat distinct from that of pesticides. While pesticides are strictly regulated under the Federal Insecticide, Fungicide, and Rodenticide Act (FIFRA) by the Environmental Protection Agency (EPA), adjuvants often fall into a regulatory gray area. The EPA generally considers most adjuvants as "inert ingredients" when part of a pesticide formulation. However, when sold separately, adjuvants are typically not subject to the same rigorous registration process as pesticides. Instead, they fall under the jurisdiction of state-level regulations, which can vary significantly. Some states, like California, have implemented more stringent requirements for adjuvant registration and labeling. Despite this decentralized approach, the industry has taken steps towards self-regulation, with organizations like the Council of Producers & Distributors of Agrotechnology (CPDA) offering certification programs to ensure adjuvant quality and safety. As awareness of adjuvants' importance grows, there's an ongoing discussion about the need for more comprehensive federal oversight to ensure consistency in quality, safety, and efficacy across the nation.
GLOBAL MARKET VOLUMES AND TRENDS
The global market for agricultural adjuvants has been experiencing significant growth, driven by increasing demand for improved agrochemical efficiency and sustainable farming practices. As of 2023, the global adjuvants market was valued at approximately $3.5 billion, with projections suggesting it could reach $4.7 billion by 2028, growing at a CAGR of around 6%. North America currently leads the market, accounting for about 35% of the global share, followed closely by Europe and the Asia-Pacific region. The market is seeing a shift towards environmentally friendly and multifunctional adjuvants, with particular growth in categories such as surfactants and drift control agents. The demand for adjuvants is driven by several factors:
RISING DEMAND FOR SUSTAINABLE AGRICULTURE:
Adjuvants that enhance the efficiency of agrochemicals and reduce environmental impact are increasingly in demand.
INCREASED ADOPTION OF PRECISION FARMING:
This is driving the need for advanced adjuvant formulations that can work with variable rate technologies.
GROWTH IN BIO-BASED ADJUVANTS:
With growing regulatory pressure and consumer demand for eco-friendly products, bio-based adjuvants are gaining traction.
Additionally, the trend towards integrated pest management and the growing popularity of genetically modified crops are creating new opportunities for adjuvant applications. However, challenges such as stringent regulations in some regions and the need for extensive research and development investments continue to shape the competitive landscape of the adjuvants market.
FUTURE OUTLOOK
The future of agrochemical adjuvants is poised for transformative changes, driven by technological advancements and evolving agricultural needs. The focus will likely be on developing more efficient, eco-friendly adjuvants that align with the principles of sustainable agriculture. Another promising area is the development of 'smart' adjuvants, which respond to specific environmental triggers like pH levels or sunlight, optimizing pesticide efficacy while minimizing off-target effects. The growing emphasis on sustainable agriculture is likely to spur innovation in bio-based and biodegradable adjuvants, derived from renewable sources such as plant extracts or microbial fermentation products. Additionally, the integration of adjuvant technology with precision agriculture and AI-driven farming systems could lead to highly customized, field-specific adjuvant formulations. As climate change continues to impact global agriculture, we may see the emergence of adjuvants designed to enhance crop resilience to extreme weather conditions. However, these advancements will need to navigate increasingly stringent regulatory environments and address public concerns about food safety and environmental protection. The successful adjuvants of the future will likely be those that can balance innovation with sustainability, efficacy with safety, and technological sophistication with ease of use for farmers.